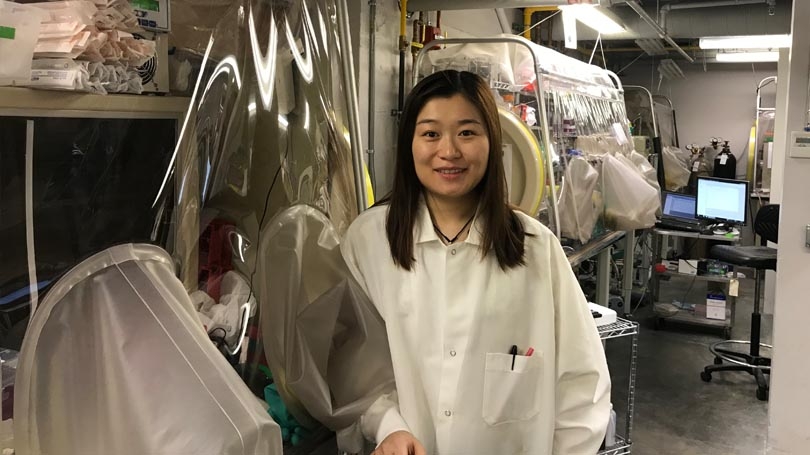
Jingxuan Cui (Lexie) in the Lynd Lab at the Thayer School (Photo: J. Cui)
To relieve rising oil demand and limit CO2emissions, renewable bioenergy has emerged as the alternative to petroleum-based fuels. First-generation bioethanol used in industry currently derived from starch (from corn) that is also used for food production. To avoid competition with food production, biofuels can be produced from the non-edible cellulose in plants (known as second-generation). One of the most efficient way to convert cellulose to ethanol at low cost is via consolidated bioprocessing (CBP).
Clostridium thermocellum, is a thermophilic (grows at 140°F) and anaerobic (will not grow in the presence of oxygen) bacterium, which has a strong native ability to consume cellulose. Its cellulose-utilizing capabilities make it a promising candidate for CBP. Wild type C. thermocellum ferments cellulose to a variety of products including organic acids, ethanol and H2.
To evaluate economic viability of a bioprocess we often use two major criteria: product yield and product titer, where yield stands for the percentage of theoretical yield of the desired product from the feedstock, and titer stands for the maximum amount of product generated from the feedstock. Metabolic engineering in C. thermocellum has been successful in increasing ethanol yield and titer, however, the maximum titer is still only 25 g/L, which is below the target of 40 g/L thought to be necessary for commercial application.
My research focuses on understanding what limits ethanol titer in C. thermocellum by investigating which part of its metabolism is inhibited by ethanol. In the Lynd Lab(led by Professor Lee R. Lynd), I have investigated this behavior using genetic modifications and enzyme assays. These are all indirect ways of determining metabolic bottlenecks. A more direct way is through the use of 13C isotopes as stable (i.e. non-radioactive) tracers of metabolic flux. An example of a 13C tracer experiment would be to add a pulse of 13C-labeled glucose to a growing culture of C. thermocellum in the presence of different concentrations of ethanol. By harvesting samples over time, I can observe the propagation of 13C-tracer from fed substrate through the various metabolic reactions that convert glucose to ethanol. If I see a high concentration of labeled substrate upstream of a particular reaction and a low concentration downstream, that would suggest that the reaction in question is the metabolic bottleneck, and will be a target for subsequent experiments. In order to perform an 13C labeling experiment, I need access to a laboratory with a high mass-resolution spectrometer (Liquid chromatography-mass spectrometry, LC-MS).
With support from the Graduate Alumni Research Award, I was able to travel to our collaborator’s lab at the University of Wisconsin, Madison(UWM), an expert in 13C flux analysis with an LC-MS/MS instrument for measuring concentrations of 13C labeled metabolites. I spent several months there, conducted 13C labeled experiments, and measured intermediate metabolite concentrations over time.
To study ethanol inhibition, 30 g/L ethanol was added in my assay before start, while using 13C-Glucose as the substrate. In the experiment, I observe the13C-tracer propagation all the way down the metabolic pathway. GAPDH is an important reaction of glycolysis, and in my experiments, compared to the no ethanol control group, we saw accumulation of the substrate and decrease of the product of the GAPDH reaction, suggesting the GAPDH reaction is a metabolic bottleneck for ethanol production. NADH is a cofactor of glycolysis and ethanol pathway, we also found significant accumulation of NADH with ethanol addition. We are still actively studying the ethanol inhibition with our collaborator, and we are expecting more results to come in a few months.
My research provides an alternative to traditional metabolic engineering strategies to study product inhibition in bacteria. Current data suggests that we should focus on improving the GAPDH reaction and find ways to resolve NADH accumulation in the future. More importantly, the approach I developed is highly applicable, it can be used in other pathways, such as syngas or butanol (other biofuels) production pathways, as well. With this approach, we can identify metabolic bottlenecks easier and faster, and it will offer us a lot more guidance on the future engineering of C. thermocellum to produce biofuels (not limited to ethanol) at a better capacity. Thus, we foresee a future using C. thermocellum as a renewable energy source that produces cleaner fuels, relieves oil demand, and reduces CO2emissions.